How to Reduce 1H NMR – Quantitative Evaluation Errors
The integration of NMR spectra can be carried out with high accuracy, but this is only possible if a number of sources of error are properly handled. On a modern spectrometer accuracy of ±5% can be achieved easily if relaxation issues are handled properly. To get errors of <1% a number of factors have to be considered and optimized.
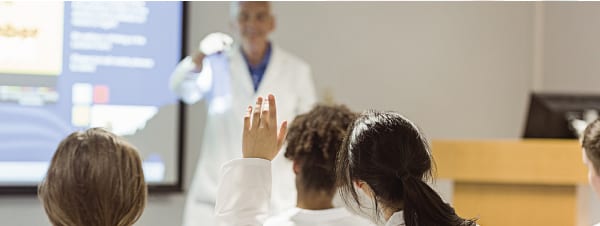
- Signal to noise
- Saturation effects
- Line shape considerations
- Digital resolution
- Isotopic satellites
- Spinning sidebands
- Baseline slant and curvature
Signal to Noise.
The spectrum must have adequate signal to noise to support the level of accuracy required for the NMR spectroscopy experiment. This means using more scans if necessary.
Saturation Effects.
NMR spectroscopy is unique among spectroscopic methods in that that the relaxation processes are relatively slow (on the order of seconds or tenths of seconds), compared to ms, us, and pico-seconds for IR and UV. In other words, once the spectrometer has perturbed the equilibrium population of nuclei by pulsing at the resonance frequency, it takes from 0.1 to 10s of seconds for them to return to their original populations. Typically, one measures the T1 (spin-lattice relaxation time1) to calculate an appropriate relaxation delay. If the pulse angle and repetition rates are too high then spectra can become saturated. Because the relaxation rates of various protons in the sample are different, integrations become less accurate. Saturation effects are particularly severe for small molecules in mobile solvents, because these typically have the longest T1 relaxation times.
To get reliable integrations the NMR spectrum must be acquired in a way that saturation is avoided. It is not possible to tell whether a spectrum was run appropriately simply by inspection, it is up to the operator to take suitable precautions (such as putting in a 5-10 second relaxation delay between scans) if optimal integrations are needed. Fortunately, even a proton spectrum taken without pulse delays will usually give reasonably good integrations (say within 3%). It is important to recognize that integration errors caused by saturation effects will depend on the relative relaxation rates of various protons in a molecule. Errors will be larger when different kinds of protons are being compared (such as aromatic CH to a methyl group), than when the protons are similar or identical in type (e.g. two methyl groups).
Line Shape Considerations.
NMR signals in an ideally tuned instrument are Lorenzian in shape, so the intensity extends for some distance on both sides of the center of the peak. Integrations must be carried out over a sufficiently wide frequency range to capture enough of the peak for the desired level of accuracy. Thus, if the peak width at half height is 1 Hz, then an integration of ±2.3 Hz from the center of the peak is required to capture 90% of the area, ±5.5 Hz for 95%, ±11 Hz for 98% and ±18Hz for >99% of the area. This means that peaks that are closely spaced cannot be accurately integrated by the usual method, but may require line-shape simulations with a program like NUTS to accurately measure relative peak areas.
Digital Resolution.
A peak must be defined by an adequate number of points if an accurate integration is to be obtained. The errors introduced are surprisingly small, and can reach 1% if a resonance with a width at half height of 0.5 Hz is sampled every 0.25 Hz.
Isotopic Satellites.
All C-H signals have 13C satellites located ± JC-H/2 from the center of the peak (JC-H/2 is typically 115-135 Hz, although numbers over 250 Hz are known) Together these satellites make up 1.1% of the area of the central peak (0.55% each). They must be accounted for if integration at the >99% level of accuracy is desired. Larger errors are introduced if the satellites from a nearby very intense peak fall under the signal being integrated. The simplest method to correct this problem is by 13C decoupling, which compresses the satellites into the central peak. A number of other elements have significant fractions of spin ½ nuclei at natural abundance, and these will also create satellites large enough to interfere with integrations. Most notable are 117/119Sn, 29Si, and 77Se.
There is a bright side to 13C satellites: they can be used as internal standards for the quantitation of very small amounts of isomers or contaminants, since their size relative to the central peak is accurately known.
Spinning Sidebands.
These can appear at ± the spinning speed in Hz in spectra run on poorly tuned spectrometers and/or with samples in low-quality tubes. They draw intensity from the central peak. SSBs are rarely significant on modern spectrometers.
Baseline Slant and Curvature.
Under some conditions spectra can show significant distortions of the baseline, which can interfere with obtaining high-quality integrations. Standard NMR work-up programs (like NUTS) have routines for baseline adjustment.
References:
- Reich, Hans J. “8.1 Relaxation in NMR Spectroscopy.” 8.1 Relaxation in NMR Spectroscopy, University
of Wisconsin, 7 Aug. 2017, www.chem.wisc.edu/areas/reich/nmr/08-tech-01-relax.htm. - Reich, Hans J. “5-HMR-3 Spin-Spin Splitting: J-Coupling.” 5-HMR-3 Spin-Spin Splitting: J-Coupling, University of Wisconsin, 10 Aug. 2017, www.chem.wisc.edu/areas/reich/nmr/05-hmr-03-jcoupl.htm#05-hmr-03-jcoupl-c13satellite.